The Board of Directors of The McKnight Endowment Fund for Neuroscience is pleased to announce it has selected ten neuroscientists to receive the 2023 McKnight Scholar Award. This is the first year McKnight makes these awards under the program’s new guidelines, which place additional emphasis on increasing diversity, equity, and inclusion to enhance the excellence and impact of our work.
The McKnight Scholar Awards are granted to young scientists who are in the early stages of establishing their own independent laboratories and research careers and who have demonstrated a commitment to neuroscience. Since the award was introduced in 1977, this prestigious early-career award has funded more than 260 innovative investigators and spurred hundreds of breakthrough discoveries.
“The committee is delighted to congratulate an array of splendid new Scholars,” said Richard Mooney, PhD, chair of the awards committee and George Barth Geller Professor of Neurobiology at the Duke University School of Medicine. “Each is committed to solving the most fundamental problems in neuroscience, from identifying the molecules that build a nervous system to decrypting the neural computations that enable us to see, learn new skills, and even form social bonds.”
Each of the following McKnight Scholar Award recipients will receive $75,000 per year for three years.
There were 56 applicants for this year’s McKnight Scholar Awards, representing the best young neuroscience faculty in the country. Faculty are eligible for the award during their first four years in a full-time faculty position. In addition to Mooney, the Scholar Awards selection committee included Gordon Fishell, Ph.D., Harvard University; Mark Goldman, Ph.D., University of California, Davis; Kelsey Martin, M.D., Ph.D., Simons Foundation; Jennifer Raymond, Ph.D., Stanford University; Vanessa Ruta, Ph.D., Rockefeller University; and Michael Shadlen, M.D., Ph.D., Columbia University.
The schedule for applications for next year’s awards will be available in August. For more information about McKnight’s neuroscience awards programs, please visit the Endowment Fund’s website.
ስለ አይኬኪርዝ የተፈጥሮ ገንዘብ ነርቭ ድርጅት
The McKnight Endowment Fund for Neuroscience is an independent organization funded solely by The McKnight Foundation of Minneapolis, Minnesota, and is led by a board of prominent neuroscientists from around the country. The McKnight Foundation has supported neuroscience research since 1977. The Foundation established the Endowment Fund in 1986 to carry out one of the intentions of founder William L. McKnight (1887-1979). One of the early leaders of the 3M Company, he had a personal interest in memory and brain diseases and wanted part of his legacy used to help find cures. In addition to the Scholar Awards, the Endowment Fund makes grants to scientists working to apply the knowledge achieved through translational and clinical research to human brain disorders though the McKnight Neurobiology of Brain Disorders Awards.
2023 McKnight Scholar Awards
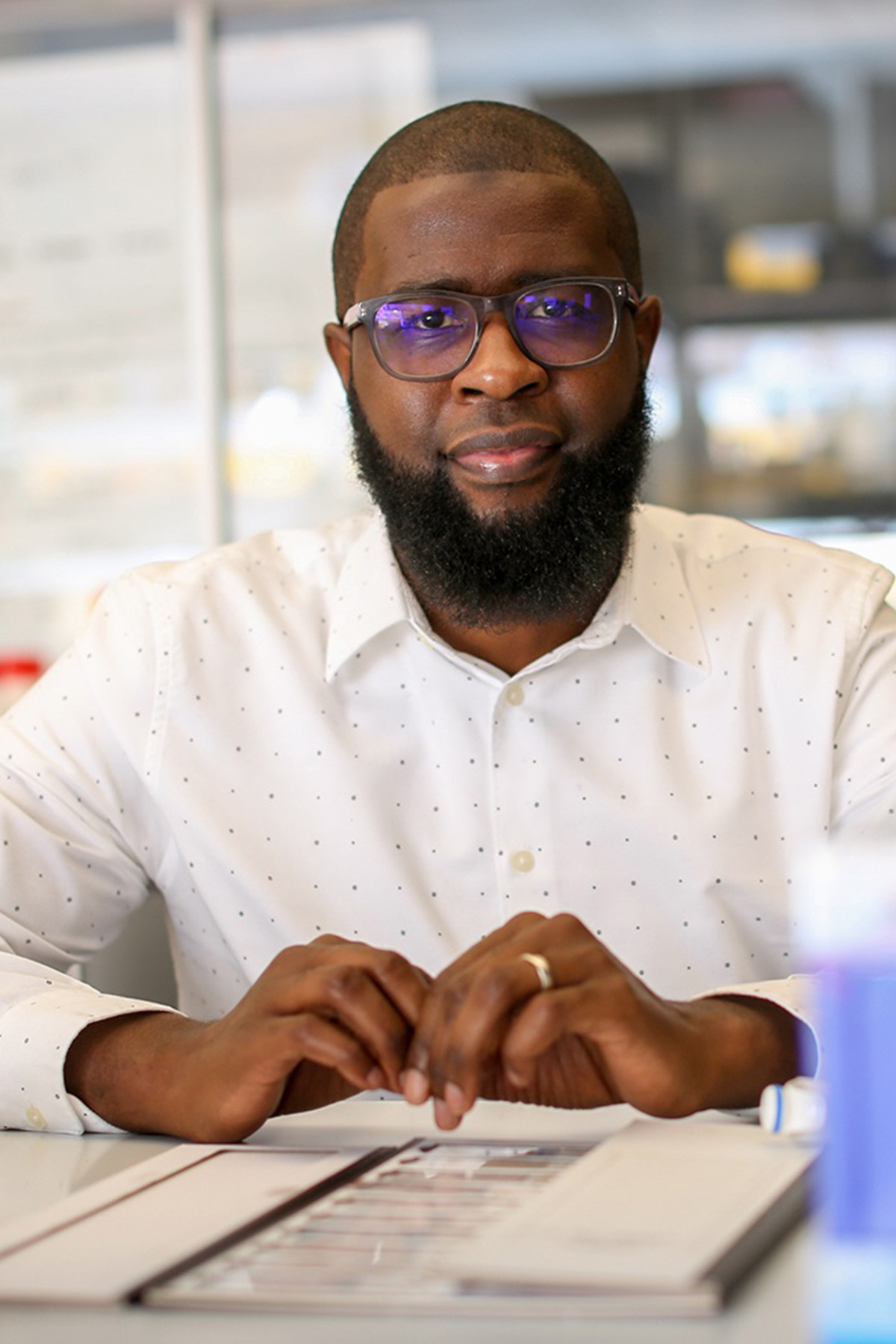
Ishmail Abdus-Saboor, Ph.D., Assistant Professor, Biological Sciences and the Zuckerman Mind Brain Behavior Institute, Columbia University, New York, NY
Skin-Brain Axis for Rewarding Touch Behaviors
Social touch is a key stimulus that is foundational to human experiences ranging from nurturing others and building social bonds to sexual receptivity. Working with a mouse model and optogenetics, Abdus-Saboor’s previous research has shown that there are direct connections between skin neural cells and the brain, and that dedicated cells are specifically tuned to certain touch cues. These cells are necessary and sufficient to elicit specific physical responses – activating the cells caused mice to respond as if they received a touch related to mating, even with no other mouse present; and deactivating them led to a decrease in response, even when paired with a social interaction.
In his new research, Abdus-Saboor and his team aim to define how neurons in the skin trigger unique positive signals in the brain, and how the brain receives and processes those signals as rewarding, as well as identifying touch neurons that are required in different touch scenarios (nurturing pups vs. grooming or play). A third aim will seek to identify what sensor on these cells identifies touch. The research will reveal more about the skin-brain connection, with potential applications for researchers studying social disorders.
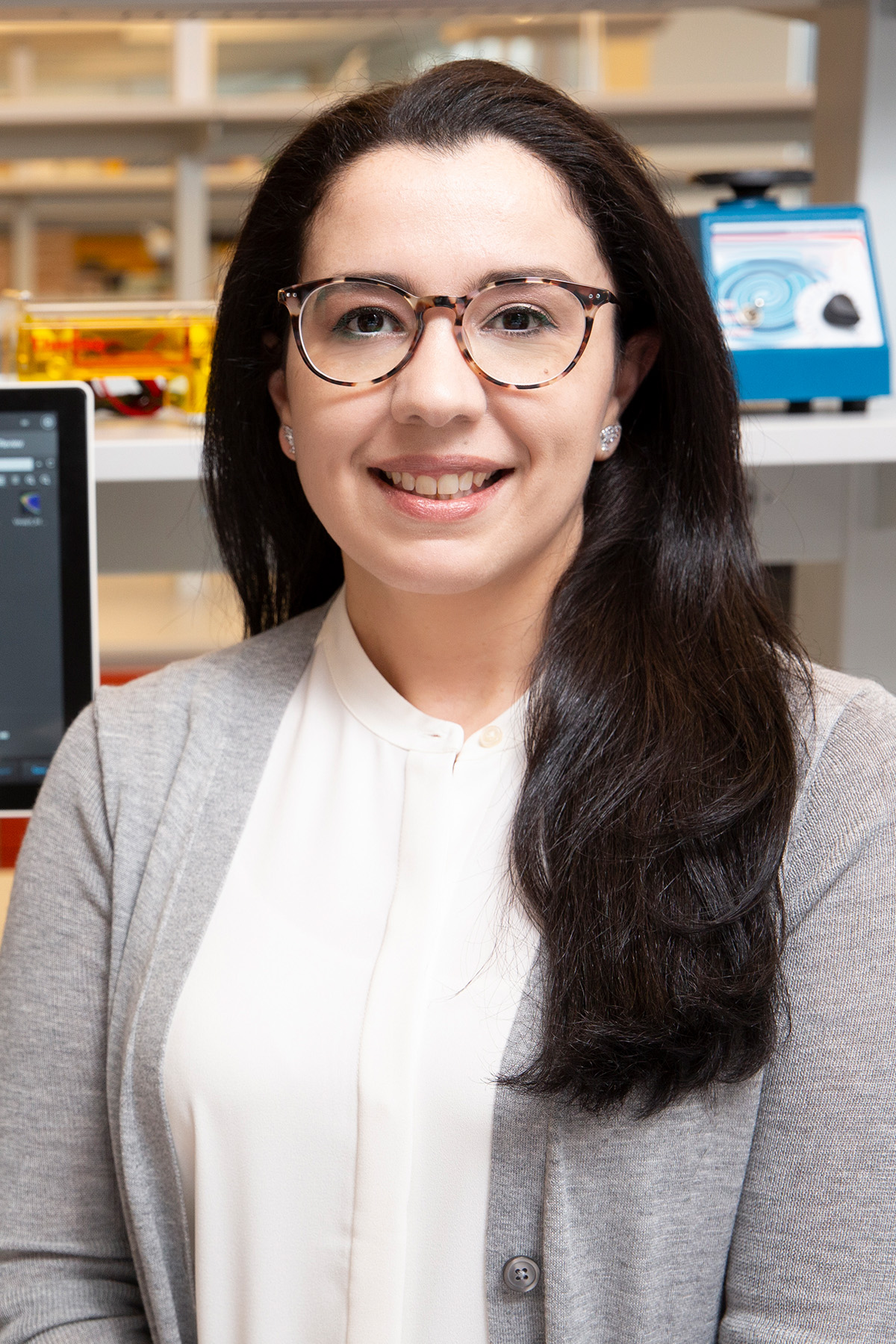
Yasmine El-Shamayleh, Ph.D., Assistant Professor, Department of Neuroscience & Zuckerman Mind Brain Behavior Institute, Columbia University, New York City, NY
Cortical Circuits for Perceiving Visual Form
In primates, roughly 30% of the cerebral cortex is dedicated to processing visual information. Using new techniques, Dr. El-Shamayleh is working toward developing a detailed mechanistic understanding of how the brain detects and recognizes the objects we see. Focusing on cortical area V4, El-Shamayleh’s research is revealing how various types of neurons in this brain region support our ability to perceive the shape of visual objects.
Cortical area V4 is highly attuned to the shape of objects in the world. Individual neurons in this area are specialized for detecting various curved segments along an object’s contour: convex protrusions or concave indentations. Different ensembles of these convex- and concave-preferring neurons can detect different objects based on what combination of convex and concave contours they contain. For example, one ensemble of neurons might detect a banana whereas another might detect a pineapple. Building on these key insights and using novel applications of viral vector-based optogenetics in a primate model, El-Shamayleh is recording and manipulating the activity of specific groups of V4 neurons with unprecedented precision. This research is identifying how various types of neurons in cortical area V4 interact to process an object’s shape, and how neural activity in this area is linked to our perception of convex and concave parts of objects. Understanding these processes will unlock details about how primate brains process visual information. Moreover, the technical innovations established in this research will also facilitate future mechanistic studies of primate brain function and primate-specific behaviors.
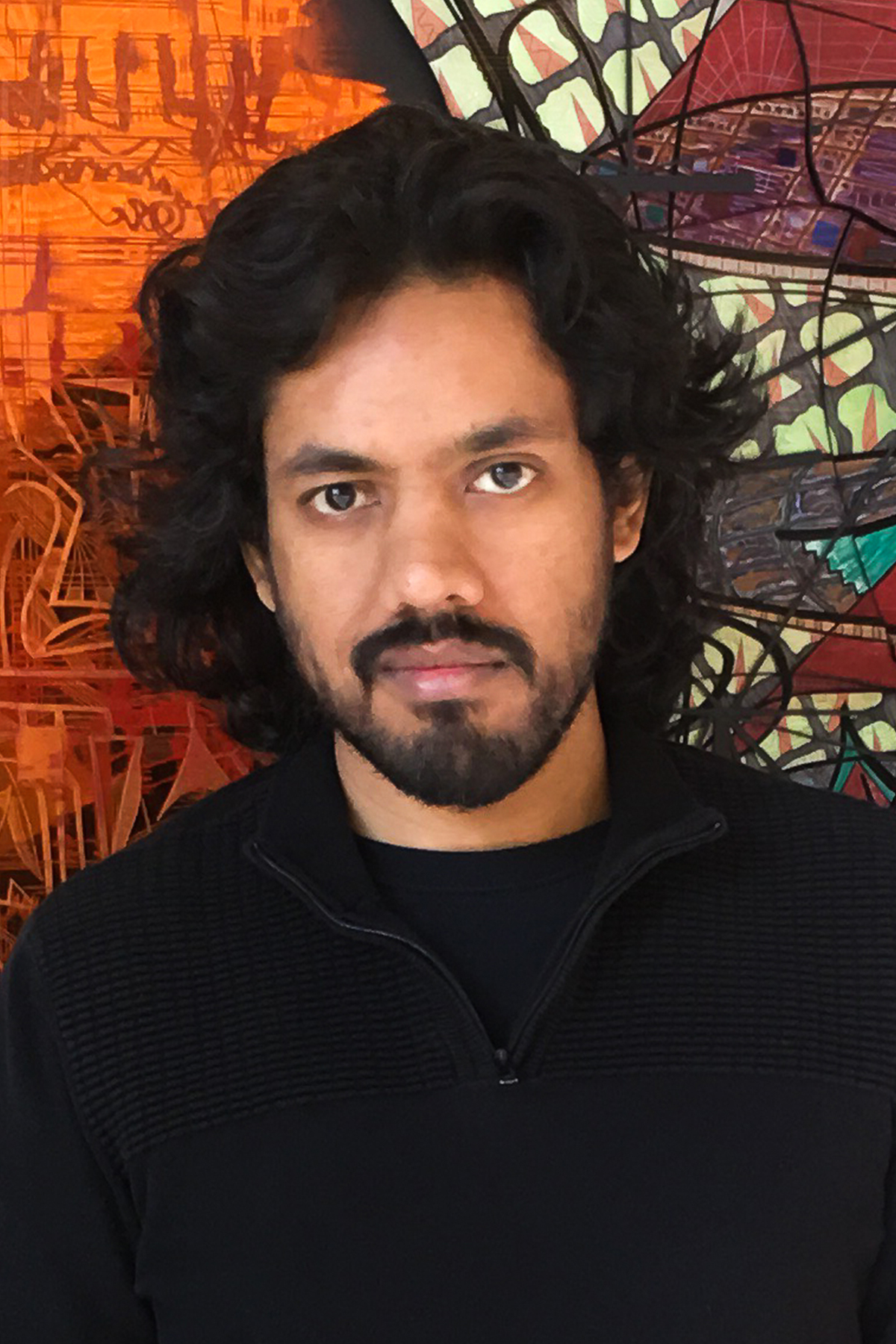
Vikram Gadagkar, Ph.D., Assistant Professor, Department of Neuroscience & Zuckerman Mind Brain Behavior Institute, Columbia University, New York City, NY
Neural Mechanisms of Courtship and Monogamy
While there has been significant research into how animals learn and perform behaviors, less attention has been paid to how one animal evaluates the performance of another during social interactions. In songbirds, most research has looked at what happens in the brains of males performing a song to attract a mate, but not what occurs in the female bird’s brain as she listens to male song. Dr. Gadagkar’s new research aims to fill this gap and create a more complete picture of these complex courtship interactions, as well as to help expand neural research to include the often-neglected female brains.
Dr. Gadagkar’s work will look at a part of the brain called HVC, a sensorimotor nucleus known to be active in males to keep time as they learn and perform their song. For the first time, he and his lab are recording what happens in female HVC as she listens and evaluates male song, to test if these neurons encode a representation of male song in her brain. Second, Dr. Gadagkar will examine how females make their evaluation, whether she compares the current performance against previous performances, and what neurons do when errors are detected. Finally, the research will look at the dopamine system to see how the brain shows a preference for the most attractive performance. This will also provide insights into the brain mechanisms of monogamy since these songbirds mate for life and use song to form and maintain their bonds.
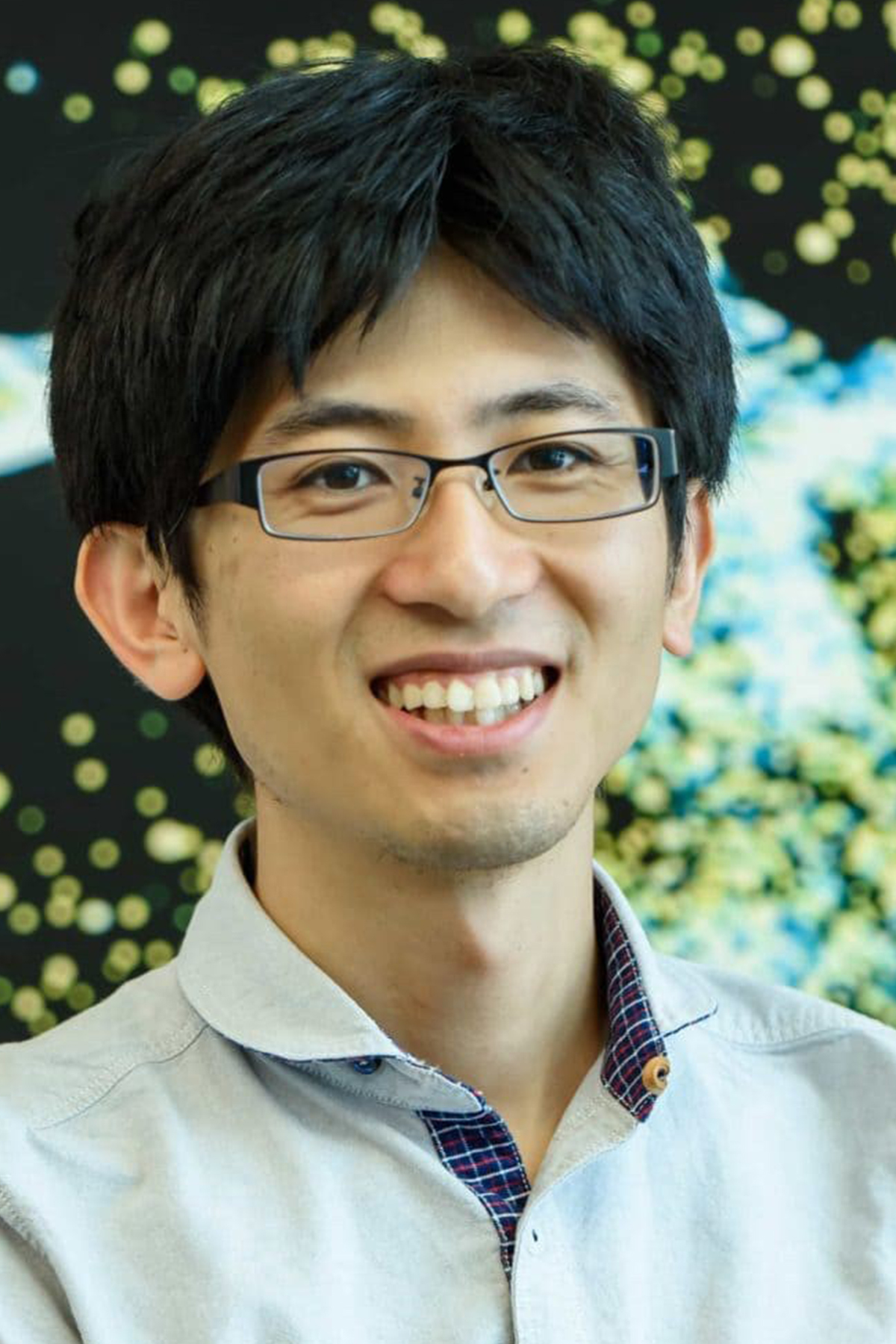
Hidehiko Inagaki, Ph.D., Max Planck Florida Institute for Neuroscience, Jupiter, FL
Synaptic Mechanisms and Network Dynamics Underlying Motor Learning
Learning a new skill requires the brain to make changes to its circuitry, a process known as plasticity. While significant research has been done to identify how brain networks execute the skill, less is understood about the mechanics of learning new skills. Dr. Inagaki and his team are working to zero in on the cells and processes involved during the process of learning. Research has shown that planned motion is controlled across brain areas, and that expert animals have different connections than novice animals. But how did those connections get there?
Using in vivo 2-photon imaging and large-scale electrophysiology in a mouse model, Dr. Inagaki and his team can now watch at the cellular level what changes are happening as a new skill is learned – in this case, learning a new timing for the action. They have observed the activity in the brain changes as animals learn to move at different timing after a cue, and seeing how those changes occur will reveal much about the mechanics of the learning process. Using genetic manipulation to enable the researchers to activate or inhibit proteins associated with plasticity, they aim to uncover not just what changes in the brain, but how those changes are initiated and consolidated. Observing behavioral changes in the animals will allow the team to link what is happening at the cellular level with our amazing ability to learn and maintain skills. Understanding more about how learning works could have implications for research into learning impairments.
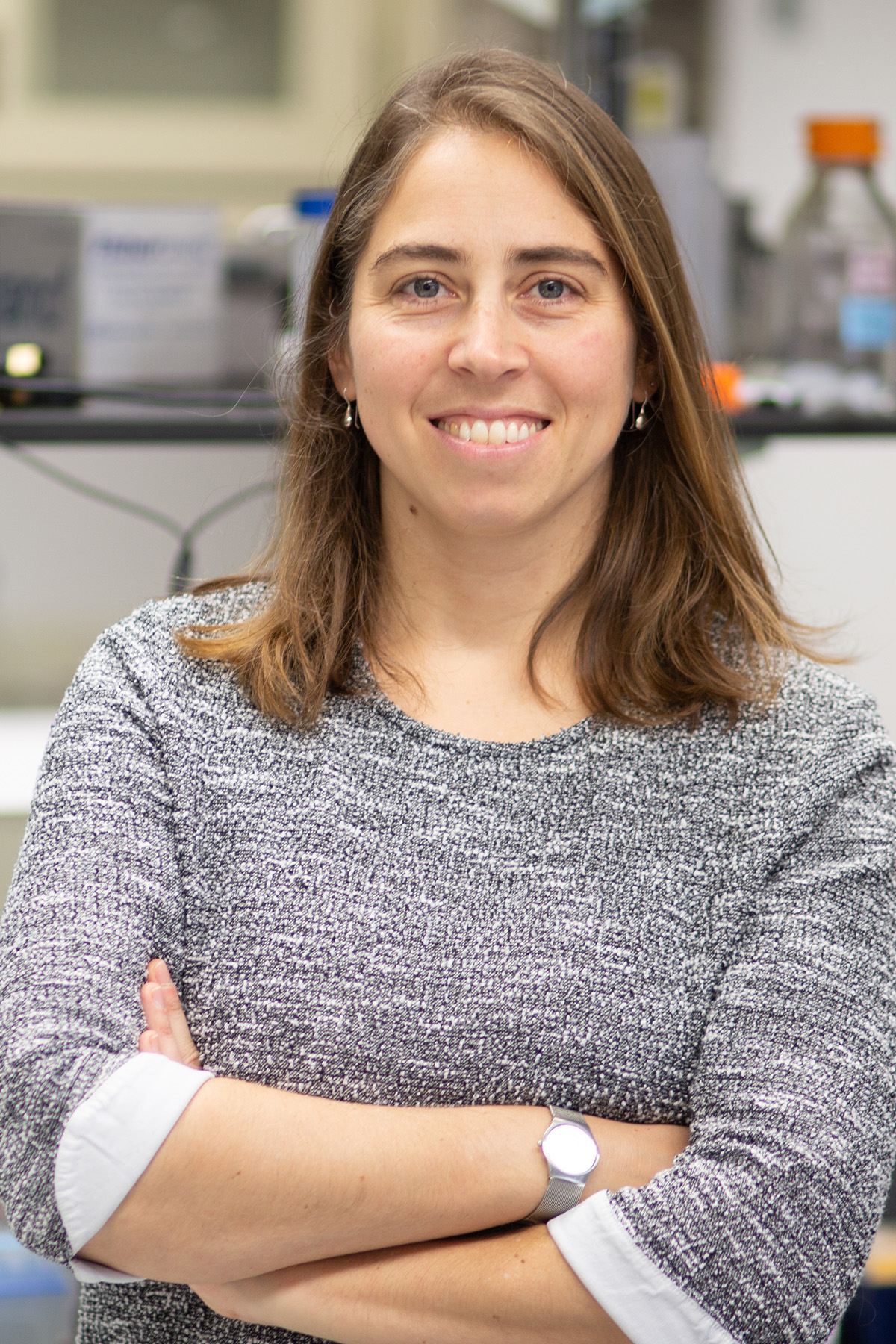
Peri Kurshan, Ph.D., Assistant Professor, Albert Einstein College of Medicine, Bronx, NY
Unravelling The Mechanisms of Synapse Development, From Molecules to Behavior
Synapses, the places where signals are sent and received between neurons, are the key to the function of neural circuits that underlie behavior. Understanding how synapses develop at the molecular level and how synaptic development influences behavior is the aim of Dr. Kurshan’s research. The dominant model holds that a class of proteins called synaptic cell-adhesion molecules (sCAMs) initiate the process, with a family of sCAMs called neurexins, which are highly associated with neurodevelopmental disorders such as autism, especially indicated. But in vivo research shows that knocking out neurexins does not eliminate synapses. So how does the process work?
Dr Kurshan uses the roundworm ሐ. Elegans as a model system to figure this out. Her work indicates that presynaptic cytosolic scaffold proteins may self-associate with the cell membrane, and then subsequently recruit neurexins to stabilize synapses. In her new research, using imaging, proteomics, computational modeling, and transgenic manipulation, she and her lab aim to identify what proteins and cell-membrane components are involved and how they interact. A further aim looks at different variants of neurexin (short and long) to see what their roles are, and how their loss leads to circuit and behavioral defects. The research has implications for a range of neurological disorders that are tied to synaptic defects.
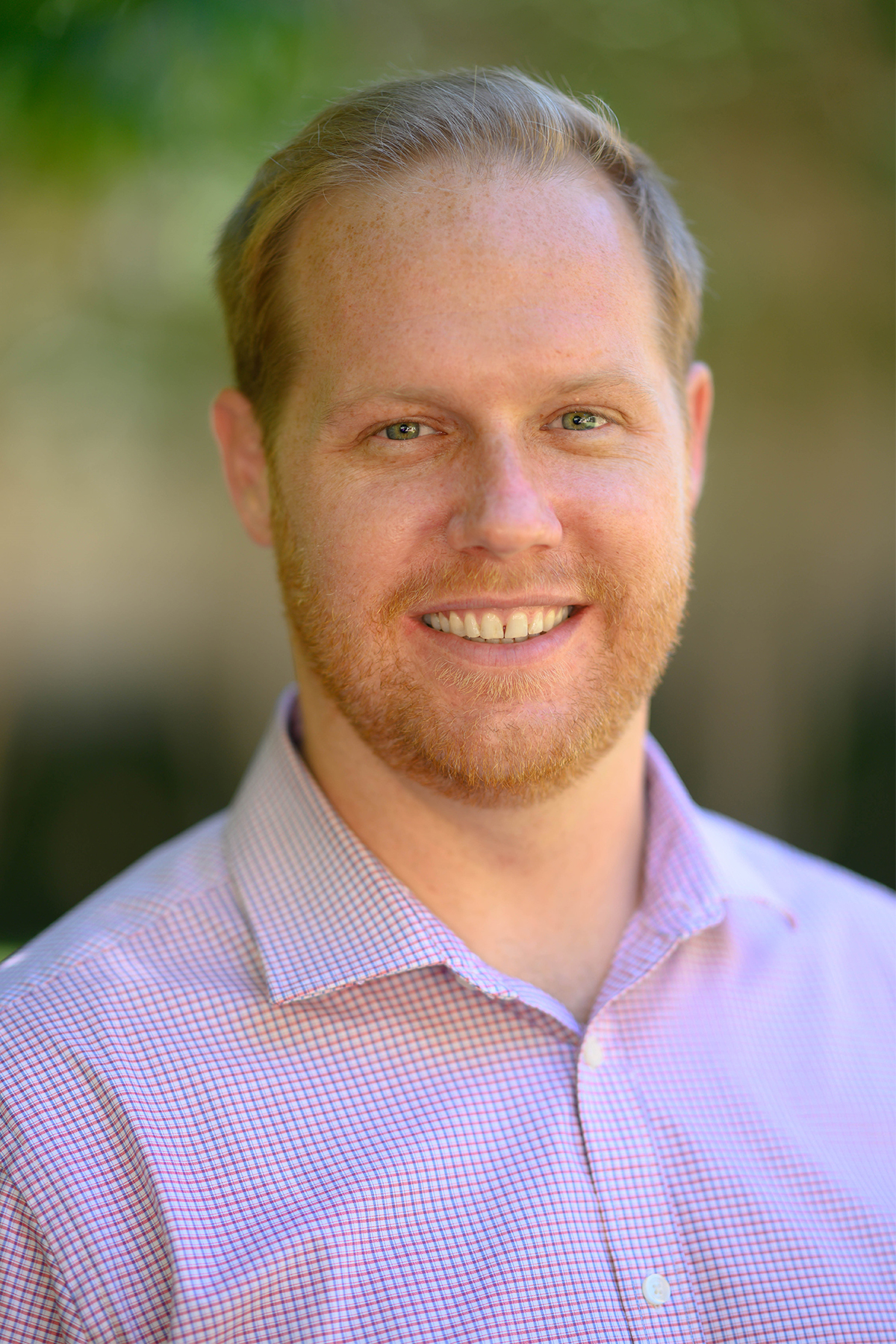
Scott Linderman, Ph.D., Assistant Professor, Statistics and Wu Tsai Neurosciences Institute, Stanford University, Stanford, CA
Machine Learning Methods for Discovering Structure in Neural and Behavioral Data
Dr. Linderman’s contributions to neuroscience lie not in laboratory experiments or making neural recordings, but in developing machine learning methods that can manage and extract insights from the staggering amounts of data these kinds of research produce. With modern technology, researchers are capturing high-resolution recordings of large numbers of neurons across the brain and simultaneously observing behaviors of freely behaving animals over long timeframes. Linderman and his team partner with research labs to develop probabilistic machine learning methods to find patterns in all that data.
Linderman’s lab is focused specifically on computational neuroethology and probabilistic modeling – essentially, figuring out how to construct and fit statistical models to the kind of data researchers produce today. His ongoing and future projects demonstrate the breadth of ways machine learning can be applied to neural research: one project looks at the impact of dopamine release on behavior, another on comparing the neural and behavioral effects of the neuromodulator serotonin, and a third on studying lifelong video recordings of freely behaving African turquoise killifish – the kinds of data that by sheer volume and complexity researchers can’t effectively parse using traditional methods. Linderman approaches the work as an integrated partner with experimental collaborators, and by developing methods to solve the problems of neurobiology is also helping advance the fields of statistics and machine learning.
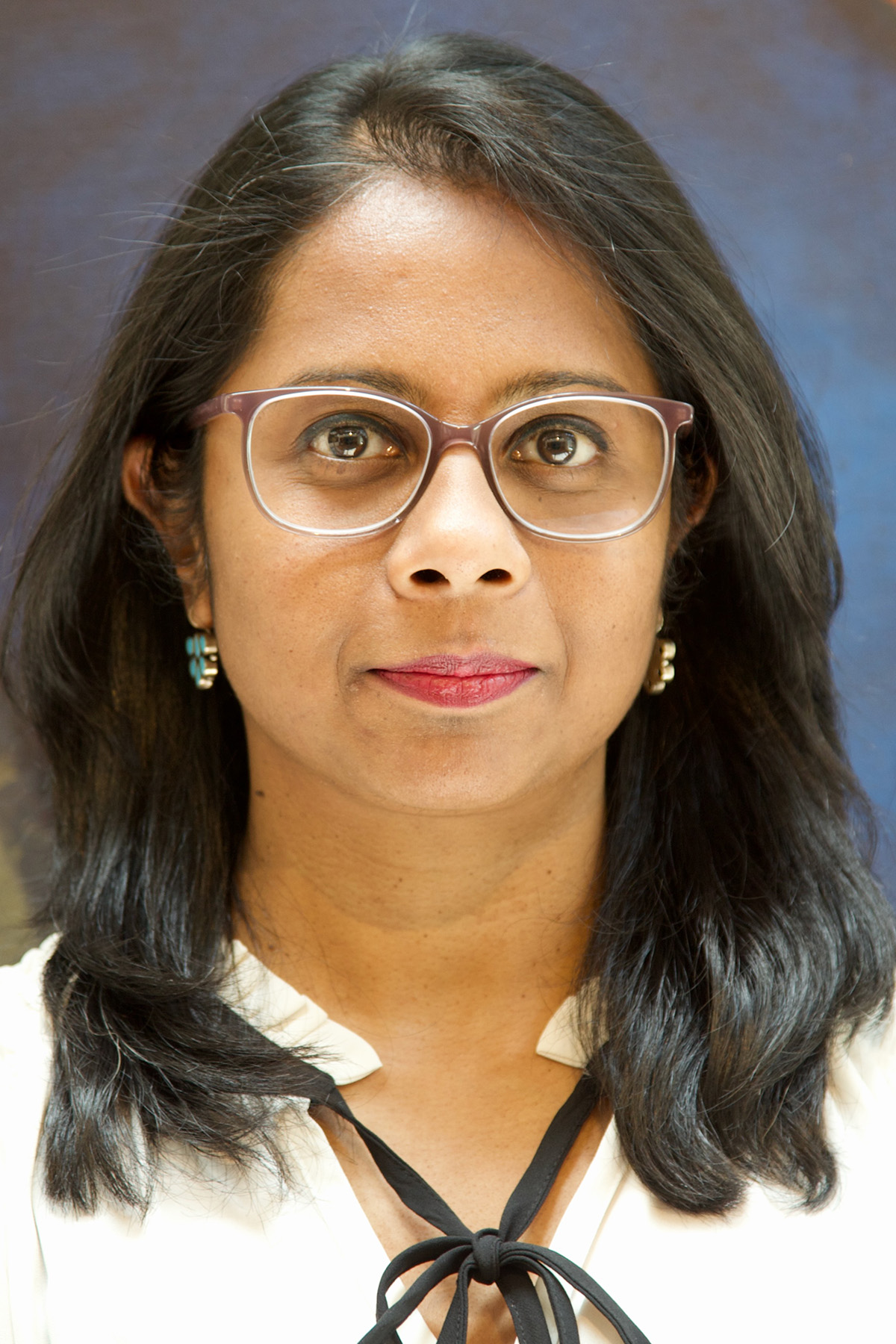
Swetha Murthy, Ph.D., Assistant Professor, Vollum Institute, Oregon Health and Science University, Portland, OR
Mechanosensation for Guiding Cellular Morphology
Mechanosensation – or the detection of physical force by a cell or a neuron – is a surprisingly subtle and multi-purpose function mediated by certain ion channels (among other proteins) on the cellular membrane. An obvious example is the sense of touch – neurons can detect pressure, stretching, and more. Dr. Murthy’s lab is digging into a much smaller-scale instance of mechanosensation with profound implications for neural health: The process of myelination, in which specialized cells called oligodendrocytes (OLs) form a sheath around a nerve to improve conduction.
It is hypothesized that mechanical cues (among other factors) can govern OL morphology and myelination, but the underlying mechanisms have remained unknown. Murthy’s lab is studying the mechano-activated ion channel TMEM63A, which is expressed in OLs, to reveal how these channels could mediate myelination and in turn shed light on how mechanical cues guide the process. Using in vitro patch-clamp techniques and genetic manipulation, Murthy will confirm OL mechanosensitivity and whether it is mediated by TMEM63A, then evaluate the dependence of myelination on TMEM63A by comparing mouse brains at different points in their development that have or have not had TMEM63A genes silenced. Finally, in vivo experiments using zebra fish will observe and document myelination in real time and determine the dependence of this process on TMEM63A. Understanding how myelination can work – and how it can fail – will be helpful to researchers studying a range of conditions tied to myelination like hypomeylinating leukodystrophies, as well as expanding the understanding of mechanosensation.
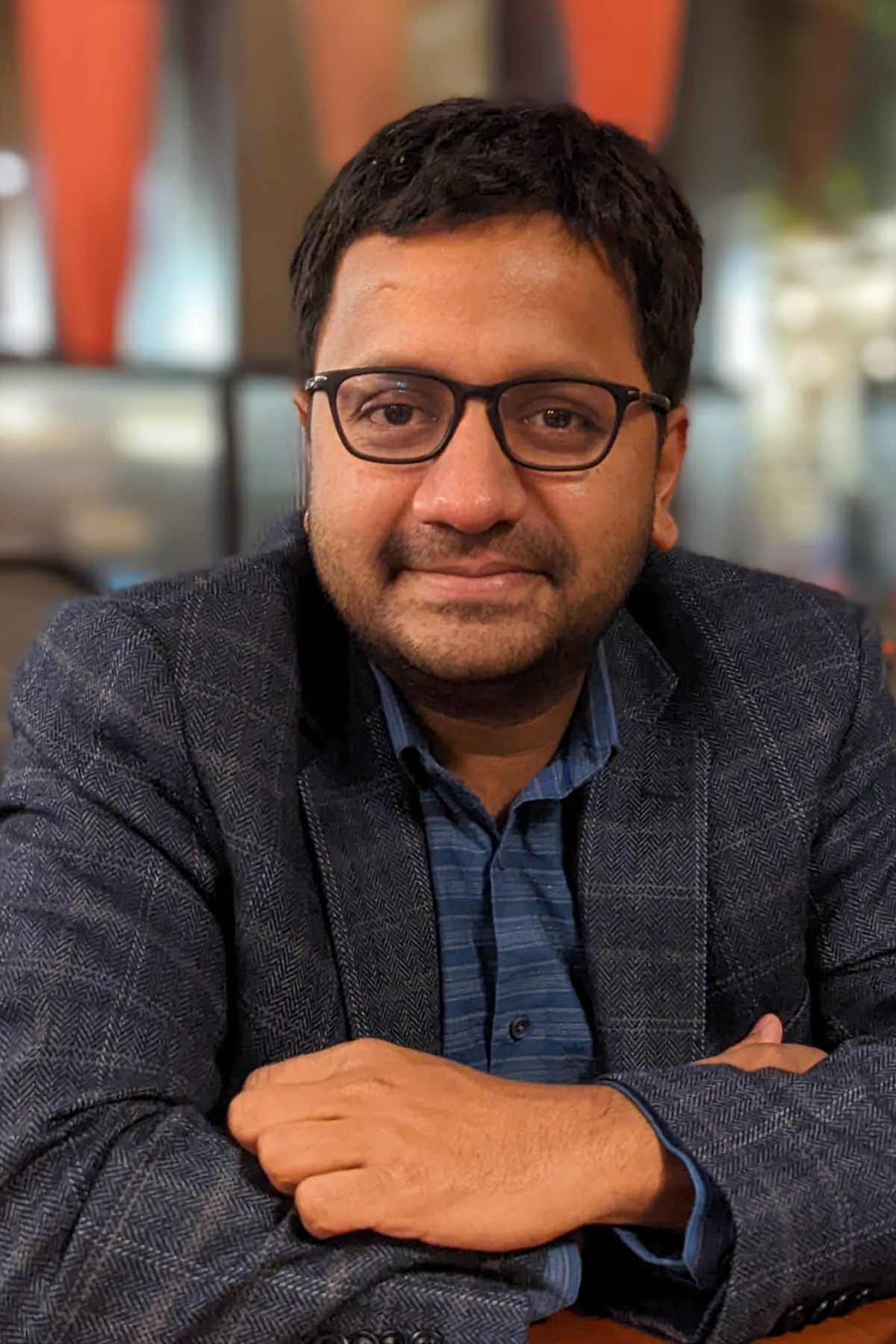
Karthik Shekhar, Ph.D., Chemical and Biomolecular Engineering/ Helen Wills Neuroscience Institute, University of California, Berkeley, Berkeley, CA
Evolution of Neural Diversity and Patterning in the Visual System
Dr. Shekhar’s lab seeks to understand how diverse neural types and their organization evolved to serve the needs of different animals. His research focuses on the visual system of the brain, specifically the retina and the primary visual cortex, which are remarkably well conserved across species separated by hundreds of millions of years of evolution. By understanding the neuronal composition in the retinas of different species, and how those neurons are organized, he hopes to uncover how evolution has acted to accommodate distinct visual requirements – and furthermore, uncover the genetic underpinnings of neural network and brain evolution.
Shekhar’s research will examine the evolutionary conservation and divergence of neuronal types in the retina of several vertebrate species, from fish to birds to mammals, and use computational approaches to reconstruct the evolution of neural diversity. He will examine if evolution led to the rise of new types or modification of existing types, including changes in morphology, function, or connectivity. A concurrent effort will investigate the visual cortex, a structure common to all mammals, and will focus on tracing the origins of early developmental epochs known as “critical periods”, where neural networks in the brain show exquisite plasticity to sensory experience. The research will help show how evolutionary adaptations occurred in the visual system, which will also point the way for further research into how other parts of the brain evolved. A guiding principle underlying Shekhar’s approach is that interdisciplinary collaborations – with engineers, neuroscientists, clinicians, and computational scientists – can bring new approaches to tackle some of the big questions in neuroscience.
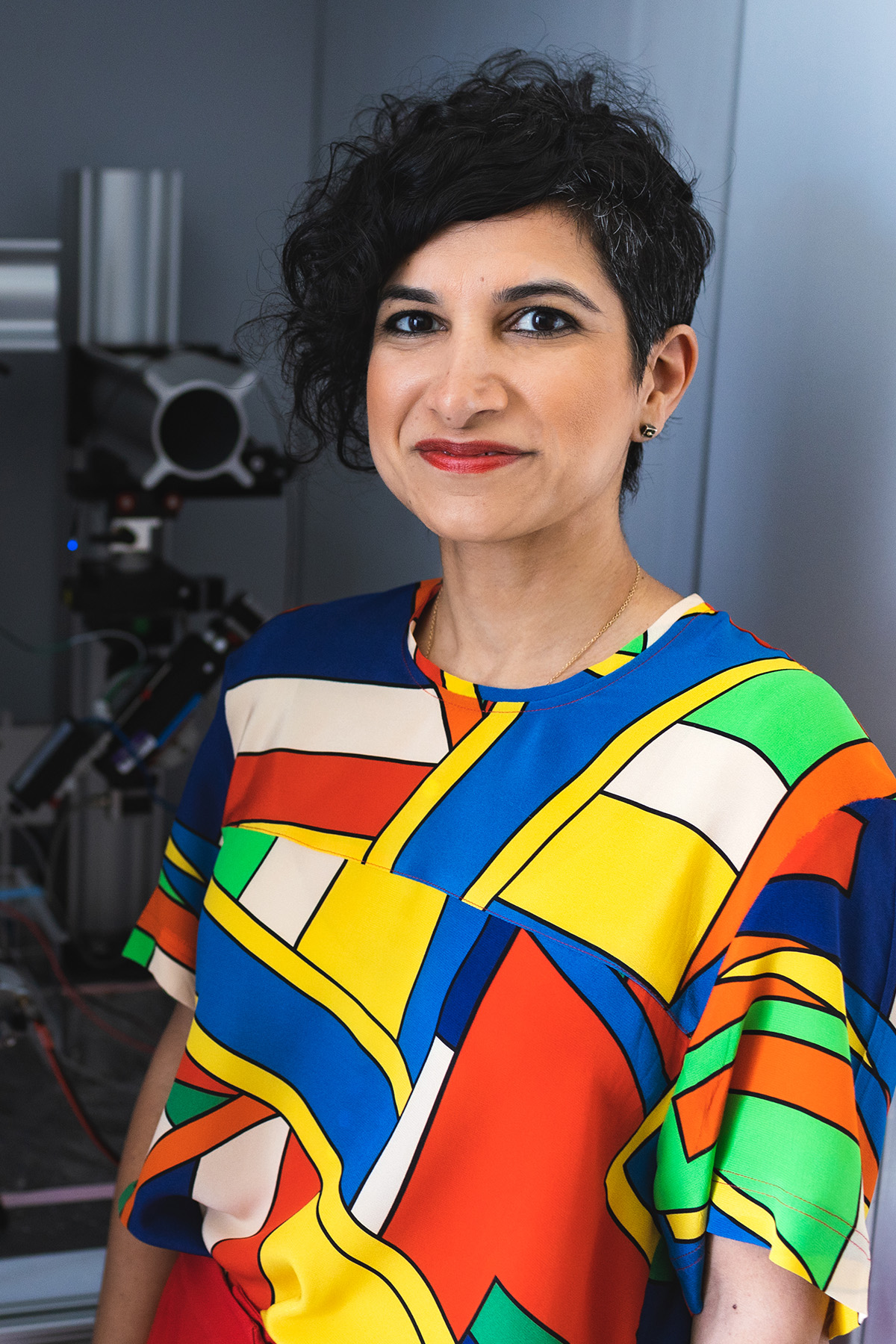
Tanya Sippy, Ph.D., Assistant Professor, New York University Grossman School of Medicine, New York City, NY
Modulation of Striatal Cells and Synapses by Dopamine Movement Signals
Dopamine is perhaps the most widely known neuromodulator, largely due to the role it plays in signaling reward. However, dopamine also plays a key role in movement, which is clearly demonstrated by the inability of patients with Parkinson’s Disease, a disorder of dopamine, to initiate movements. Dr. Sippy aims to help learn more about how dopamine is involved in movement, through very precise in vivo measurements of dopamine fluctuations simultaneously with the membrane potential in target neurons.
Membrane potential recordings allow Dr. Sippy’s lab members to measure two properties of neurons that are known to be affected by neuromodulation: 1) the strength of synaptic inputs and 2) the excitability of the neurons that determines how they respond to these inputs. But measuring both dopamine fluctuations and membrane potential in one cell is very hard. Sippy’s work hinges on the discovery that dopamine activity is mirrored in the two hemispheres of the brain, and so measurement of it and membrane potential can be made on opposite sides and still have strongly correlated results. With these recordings made, Sippy will optogenetically manipulate the dopamine system and see how activating or suppressing dopamine affects the properties of target neurons, and how this affects the actions of the animal.
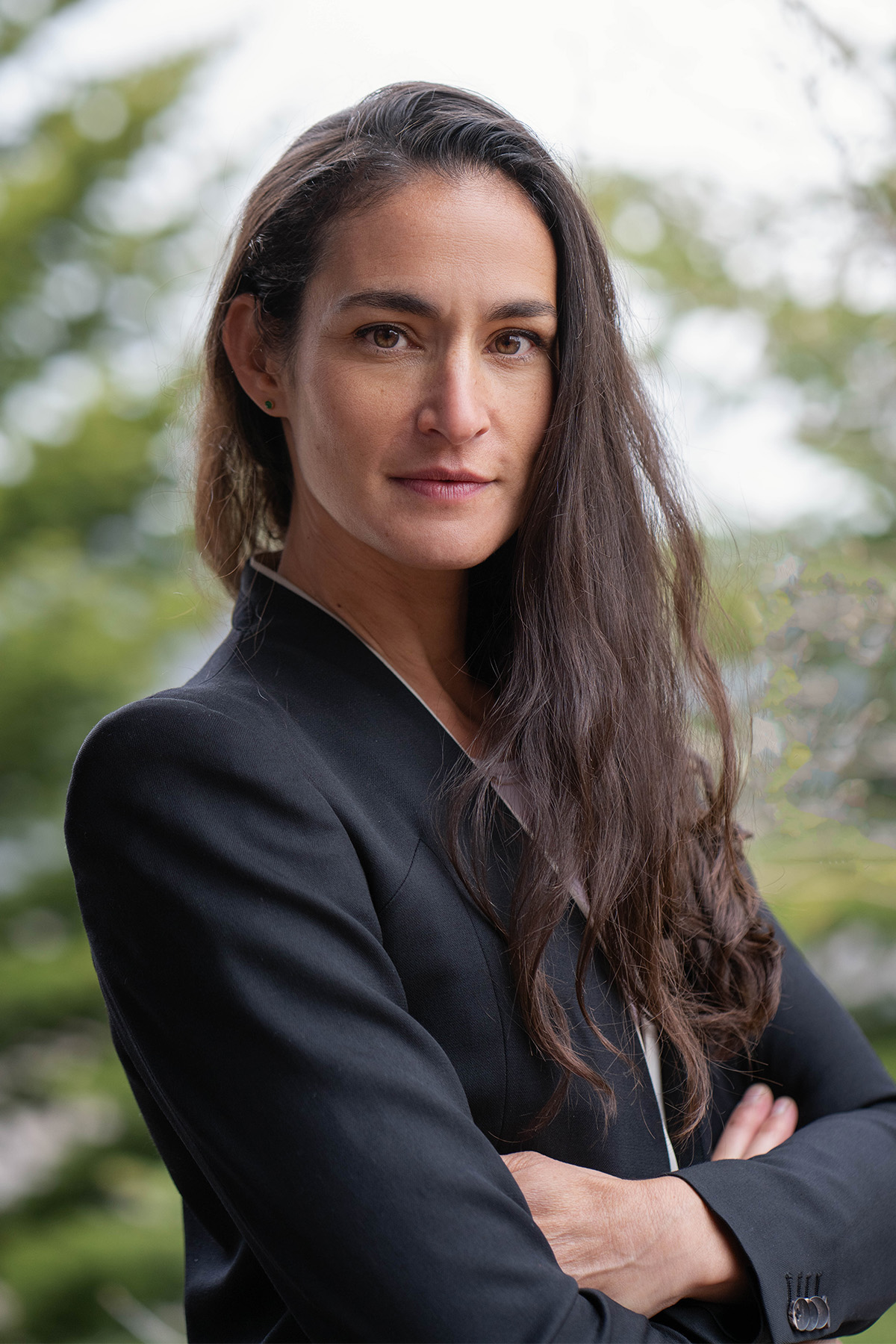
Moriel Zelikowsky, Ph.D., Assistant Professor, University of Utah, Salt Lake City, UT
Neuropeptidergic Cortical Control of Social Isolation
Prolonged social isolation can negatively impact mammalian life – resulting in cognitive decline, heart disease, and behavioral changes, including a steep rise in aggression. While many studies have looked at subcortical control of natural forms of aggression, such as those that characterize territorial defense or protection of offspring, few have looked at pathological forms of aggression or their top-down control. Dr. Zelikowsky aims to better understand the mechanism and cortical circuits involved in the rise of aggression as a result of chronic social isolation.
Initial research using a mouse model identified a role for the neuropeptide Tachykinin 2 (Tac2) as a subcortical neuromodulator of isolation-induced fear and aggression – when Tac2 signaling was silenced, aggression was reduced in isolated mice; when activated, aggression increased even in non-isolated mice. Critically, Tac2 was also found to be upregulated in the medial prefrontal cortex (mPFC) after social isolation, however, its function in the cortex remains unknown. Further research will now examine exactly how Tac2 interneurons in the mPFC mediate aggression in socially isolated animals. The research uses cell-type specific perturbations in mice who have experienced social isolation and are exposed to encounters with same-sex “intruder” mice in their space. Machine learning is used to identify clusters of behavior, which are mapped to imaged brain activity. By understanding how isolation can change the brains of mammals, future researchers may be able to better understand the effects of extended social deprivation in humans – and how to address them.